This is a new sort of blog for us, it’s a Primer. So, a very extended, long shelf-life blog that gives you a detailed look into the issues involved in a particular transition theme. Why is so much longer than our normal “long blogs” - put simply, it needs to be. Sometimes simplifying is good, and sometimes there is no substitute for explaining something properly. Happy reading.
Why should we care about Antimicrobial Resistance (AMR)?
As a child my knees, elbows and forearms were constantly grazed. A combination of regular school sports, playground ‘sports’ and just general clumsiness. I was fortunate never to have broken a bone (other than fingers during basketball) and never had any stays in hospital. The nearest I got to an anesthetic was a jab in my foot to have a verruca removed. I had colds of course and the various childhood diseases generously distributed at ‘pox parties’.
Before the discovery of antibiotics, bacterial illnesses such as pneumonia, TB and enteritis and even simple cuts and grazes could be fatal. In 1930, almost 300,000 Americans died of bacterial illnesses (22% of all deaths that year). By 1952, by which stage antibiotics were used widely, less than 95,000 Americans died from the same bacterial illnesses (6% of all deaths). The death rate from bacterial infections per 100,000 people fell from 216 in 1930 to 59.7 by 1952.
Antibiotics revolutionised surgery and healthcare
Antibiotics and other antisepsis measures revolutionised surgery and healthcare. Highly invasive operations, such as gut surgery and joint replacements all depend on antibiotics to prevent infection and be successful. Certain treatments which render the immune system less effective, such as chemotherapy, are made viable by the support provided by antibiotics. Where previously catching certain infections was pretty much a death sentence, antibiotics and other antimicrobials provided hope.
However, microbes got wise.
Many common diseases, including respiratory and urinary tract infections and sexually transmitted diseases started to become less responsive to antibiotics. Once again simple cuts and grazes could be dangerous. In an eerie parallel with his character in the 2000 film Castaway, Tom Hanks actually did get a serious infection from a cut leading to hospitalisation and him nearly dying.
Left unchecked, AMR could become the major source of death globally.
AMR is still killing too many
A landmark 2016 report “Tackling drug-resistant infections globally,” a review of antimicrobial resistance (AMR) chaired by Jim O’Neill, estimated that 700,000 deaths per year were due to AMR. The report forecast that figure reaching 10 million by 2050, costing the global economy more than US$100 trillion and pushing millions of people into extreme poverty.
A study estimating the AMR burden in 2019 published in January 2022 in The Lancet found that 1.2 million deaths were attributable to bacterial AMR globally. That means that AMR is the leading cause of death, higher than HIV/AIDS (~up to 860,000) or malaria (627,000). The study’s co-author Professor Chris Murray from the University of Washington commenting on the O’Neill report estimate of 10 million deaths per annum by 2050 said that “...we now know for certain that we are already far closer to that figure than we thought.”
Low Income and Middle Income Countries (LIMIC) are disproportionately impacted. Children in sub-Saharan Africa are more than 14x more likely to die before the age of 5 than children in high income countries.
Antimicrobial Resistance (AMR) is a macro threat to the sustainability of the human race and other species. It is of a similar level to that of the worst impacts of climate change. Bringing together biological, behavioral, and physical solutions with appropriately incentivising funding we should be able to continue to enjoy the benefits of our microbe partners whilst avoiding their darker side. This is potentially a massive, if complex, investment theme for those who care about sustainability; the potential goes well beyond the pharma industry.
Alleviating the impact of AMR will help us hit UN SDG 3
United Nations Sustainable Development Goal 3 aims to ensure healthy lives and promote well-being for all at all ages as this is “important to building prosperous societies.” Alleviating the impact of AMR is an important part of the journey to achieving SDG 3 targets. Digging deeper into goal 3, one of the 2030 targets includes ending preventable deaths in newborns and the under fives targeting neonatal mortality at least as low as 12 per 1,000 live births (2020: 17 globally) and under-5 mortality to at least as low as 25 per 1,000 live births (2020: 37). Another target called for an end to the epidemics of AIDS, tuberculosis, malaria and neglected tropical diseases and the combating of hepatitis, water-borne diseases and other communicable diseases. There have been some advances - from new combined therapies allowing people to live with conditions such as AIDS to new vaccines for eradicating malaria, but the longevity of treatments is a risk factor.
Investors showed their concern with the formation of the Investor Action on Antimicrobial Resistance Initiative with investors representing more than US$7 trillion as founding members. They believe that the financial industry is not taking AMR seriously enough. It’s a sustainability theme where investors can make a real difference
Take a step back - what is AMR?
When microbes adapt either over the long term through genetic mutation or using existing defence mechanisms, they can resist attempts to stop them being infectious and causing disease. That adaptation is helped when antimicrobials, especially antibiotics, are over- or inappropriately used. Not only does that mean antimicrobials could become less effective at treating/preventing infections, but they may also stop working altogether.
There are some infections that defy even powerful antibiotics. You have most likely heard of a hospital superbug called MRSA - “Methicillin-Resistant Staphylococcus Aureus” (note that going forward I am going to use the shortened forms of bacteria names. In this case Staphylococcus Aureus becomes S.aureus.)
Understanding the biology of AMR
There are a couple of other questions we need to answer first to get a fuller understanding of the topic.
What is a microbe? (the “M” in “AMR”)
How do microbes interact and impact us? This gives us a clue as to how they become resistant to treatments and how we might alleviate AMR.
I am not a microbiologist (I studied engineering) so my explanations that follow are simplified for brevity and designed to help with broader understanding of the AMR topic.
What is a microbe?
A microbe is an organism that is too small to be seen with the naked eye. There are five main types which are laid out in Figure 2. They can have either a symbiotic relationship with humans (and animals and plants) where there is a mutual benefit (‘good microbes’) or a pathogenic relationship leading to disease. Probably the best-known example of the ‘good microbes’ is the gut microbiota where there is growing evidence of the benefits of maintaining the right balance for overall good health and well-being. This can be done through diet, supplements or maybe even exercise.
The eagle-eyed among you will spot that Figure 2 shows six microbes, not five. Prions are resistant to most antimicrobial methods as they don’t contain nucleic acids (DNA or RNA). I remember vividly the BSE or ‘mad cow disease’ outbreak in the UK in the late 1980s. There was concern that it could pass to humans in the form of variant Creutzfeldt-Jakob Disease (vCJD). Prion diseases are rare and so I am excluding them from this discussion.
Plants comprise the bulk of biomass on the planet but bacteria come in second making up approximately 15%, in fact 35x the biomass of ALL animals combined. So, let’s look at bacteria a bit more closely.
Bacteria in more depth
From Figure 2 you can see that bacteria are cellular, living organisms that are one of the prokaryotic groups (archaea being the other). They can be a number of different shapes and can be found either as single cells, pairs or clusters and chains.
Their DNA is a single loop and floats freely within the cell as there is no nucleus although there can sometimes be an extra loop of genetic material called a plasmid which can contain genes that, for example, confer resistance to some antibiotics. This can be passed on to following generations of bacteria or even sideways to other bacteria.
Bacteria are surrounded by a cell wall containing peptidoglycan (a polysaccharide or long chain of sugars) which protects the cell from bursting and protects it from the environment. Some bacteria have a thin peptidoglycan wall surrounded by a lipopolysaccharide membrane and are referred to as Gram-negative. Others have several layers of peptidoglycan many times thicker, but no outer membrane and are referred to as Gram-positive - named after the Danish bacteriologist Hans Christian Gram who developed a staining method for identifying each class. Gram-negative are typically harder to treat than Gram-positive that absorb antibiotics and cleaning products more readily.
Some bacteria can grow in the presence of (aerobic) or the absence of (anaerobic) oxygen. Others require it to be present or absent (obligates).
Some bacteria can also form endospores which form on the surface of bacteria with a special coating that can make the bacteria resistant to radiation, heat and other harsh environmental conditions, making them harder to destroy.
However, by far and away the majority of bacteria live within us or their hosts quite happily and symbiotically without causing infection. Indeed, they even provide benefits such as aiding digestion, providing nutrients and protecting us from other pathogens. The Lactobacillus species can be found in the gut, mouth and the female genital system, for example, happily coexisting with us. There are thought to be marginally more bacteria (and fungal and protozoan) cells in the human body than there are human cells comprising between one and three percent by mass. So how do they cause disease?
How do microbes interact with us?
As previously mentioned, most of the microbes in our bodies have a symbiotic relationship with us. Sometimes one microbe can help us deal with another. For example, bacteriophages are a type of virus that can attack bacteria. They mostly exist in our guts, and the mucus we produce in our guts even helps them in their battle (microscopic high five!)
Some bacteria which are helpful to us can become pathogenic or infectious if our immune system is weakened or they come into contact with a normally sterile area of the body.
Some are just naturally bad. How those bad microbes end up in or on our bodies is via other living organisms such as mosquitos, ticks and lice (“disease vectors”) that typically transmit via blood through bites or through inanimate objects such as food, water which is contaminated (“vehicular”) and surfaces (“fomites”). With the latter, normally the microbe finds its way onto the skin (usually the hand) and then onto a mucus membrane when touching either the face or in some cases the genitals.
Biofilms - help and hinder
If you have ever had a tooth cavity or gum infection, dental plaque was the cause. Dental plaque is an example of a biofilm - a natural accumulation of microbes, in this case bacteria, that can occur on almost any surface, including the inside and outside of the human body. Some are beneficial, helping to prevent pathogenic bacteria colonising, while others can cause health problems.
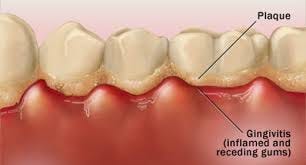
If a biofilm contains different bacterial species, antimicrobial resistance (AMR) can spread between them (remember earlier we talked about plasmids that can contain resistance genes?)
How is resistance propagated and perpetuated?
We discussed earlier the various ways in which microbes can be spread to humans and that leads us on to ways in which AMR can propagate through communities. Here are a few examples, and we shall explore more as we look at solutions later in this blog.
Water systems: There are a number of ways in which excess antibiotics can enter water systems and bacteria can, with prolonged exposure, develop resistance to those antibiotics. This can impact both humans and other animals. For example, in the Gombe National Park in Tanzania, half of the less than 100 strong chimpanzee population were found to have bacteria resistant to most common antibiotics in their faeces. The local human population had access to cheap non-prescription antibiotics (sulphonamides) and would use them without measure for treating diarrhoea. In addition, people used streams for washing, contaminating the water - water drunk by the chimpanzees and others - with both bacteria and excess antibiotics.
Pharmaceutical waste: There are two areas here. Firstly, the release of antibiotics and other pharmaceuticals into rivers as waste from the drug manufacturing process can potentially lead to new combinations of resistant genes. Secondly, standardised dosing can result in unused quantities of antibiotics and other antimicrobials being discarded at the point of delivery (hospitals, clinics and the home) into sinks, toilets and ultimately the water system.
Microplastics: It is estimated that the amount of plastic added to existing waste each year could reach 380 million tonnes in 2040, more than double the levels in 2016. There is plenty of discussion on the harmful effects of microplastics on sea and ocean wildlife and ultimately the food chain. However, there is also growing evidence that microplastics may harbour antibiotic resistant bacteria too, and a study from the New Jersey Institute of Technology estimated that certain strains of bacteria had 30 times the level of resistant genes in biofilms on microplastics than those on sand.
From mother to child: A University of Copenhagen study found that antibiotic resistant genes could be passed from mothers to children implying a link between antibiotic use by pregnant mothers and subsequent resistance in newborns. E. coli which is a commonly found bacteria in the gut seems to act as the main collector and potential spreader of antibiotic resistant genes to other gut bacteria.
Birds: Of course, animals and insects in general can be vectors for passing on resistant genes, but the distances that birds can cover singles them out. Research has shown that many bird populations carry drug resistant bacteria, possibly picked up from scavenging landfill sites, that they can spread to other species.
Pets: Finally, as well as resistant genes potentially passing between pets and their owners, and potential overuse of antimicrobials by pet owners, raw dog food which has become trendy in recent years, has been found to carry more resistant bacteria than other types of dog food. A 2019 study found almost 73% of raw dog food samples contained levels of enterobacteria (highly resistant) at levels beyond EU safe levels for pets.
How do we stop harmful microbes?
There are three main ways:
Remove the microbe (or stop it being there in the first place)
Kill the microbe
Stop it becoming pathogenic
Much in the same way that climate change does not have a single solution or pathway, to combat antimicrobial resistance, we need to attack the problem from a number of angles.
New drugs are only part of the answer
If at the high level we are “running out of antibiotics that work” the natural focus would be to “find more antibiotics.” That is a rational thought and a valid approach. It is however only one avenue - biological. We can also look at ways to reduce the chance for microbes to develop resistance by reducing the need to use them in the first place. There are behavioural changes including improving hygiene at personal and corporate levels and potentially changing how people interact to reduce the risk of spreading infection. Plus, using antimicrobials only for the appropriate infection, and reducing waste antimicrobials from entering water systems
However, through our understanding of the way in which microbes interact with us, there may be ways to disrupt them physically and stop them being pathogenic, for example stopping them forming biofilms.
Finally, as with any innovation that requires transition the right financial and political incentives need to be in place.
There are some big problems that humanity faces and often those macro risks have causal connection. For example, climate change causing thawing Arctic permafrost could release potentially fatal microbes for which we have no treatment. What is clear is that a concerted effort is needed if AMR is to be controlled.
Those involved in investing decisions can play an important part in enabling each area depicted in Figure 3, across a variety of industries from health care to agriculture to food preparation and even to fashion.
Let’s look at each in turn and explore some case studies. Subsequent blogs will deep dive into these areas.
Biological solutions
Scientists are working hard to develop new antibiotics and boost the effectiveness of existing ones.
They are also seeking alternative therapies that could be just as good, or even better than existing ones and attempting to gain a greater understanding of how bacteria work. These are mostly about “killing the microbes.”
Some examples below, but there are many more not discussed.
Novel compounds: A direct approach, as existing antimicrobials are becoming ineffective due to resistance, would be to look for new antimicrobials. There are a number of promising compounds.
MBX-4132 is an example of a new antibiotic compound that cleared a multidrug resistant strain of gonorrhoea.
Whilst most antibiotics penetrate the bacterial wall to kill them, Gram-negative bacteria are very hard to treat as it is difficult to penetrate that wall. Darobactin, which is isolated from a bacteria found in the gut of nematodes, has a shape similar to the protein that bacteria produce to build their outer membrane. So, whilst it cannot penetrate the interior it can sit and block the transport route for the bacteria’s shell components causing them to die.
Peptide Nucleic Acids (PNAs) target genetic sequences within bacteria. They can be quicker to design and can adapt more effectively to AMR than traditional small molecule antibiotics.
We know that bacteria are very good at defending themselves, sometimes against other bacteria by producing antibiotics. Remember bacteriophages? Viruses that kill bacteria. A. baumannii is the world’s leading superbug, responsible for up to 20 percent of infections in intensive care units. Phages have been used to resensitise A. baumannii to at least seven different antibiotics.
We mentioned earlier that microplastics could harbour resistant bacteria. That also opens up the possibility that we could find novel antibiotics in them too including ones that can fight antibiotic-resistant superbug strains.
Repurposing and new combinations: Using existing remedies in a different way is not new but combining different antibiotics and indeed antibiotics with other substances can improve their effectiveness against resistant bacteria.
A decades-old treatment for congestive heart failure and glaucoma, has been repurposed to be able to prevent vancomycin-resistant enterococcus (VRE) colonising in the gut. This means that antibiotics are not needed that often destroy good intestinal bacteria which in turn leaves people vulnerable to other infections.
Synthetic cannabidiol (CBD) which is already used to treat a variety of conditions including chronic pain, epilepsy and anxiety has also been shown to have antimicrobial properties. Treated hemp fibres are also effective with one study finding that even after washing 30 times the material was still almost 90 percent effective against E. coli and S. aureus.
New combinations of existing drugs with antibiotics, different antibiotics together or even “regular” drinks and antibiotics can improve performance. For example a molecule originally developed to treat Alzheimers’s, Parkinson’s and Huntingdon’s diseases called PBT2 increases S.pneumoniae’s sensitivity to the antibiotic ampicillin, or polymixin B combined with doxycycline which significantly reduces biofilm formation of P. aeruginosa all the way to this beauty - cranberry extract can make bacteria more sensitive to antibiotics or in other words make them more effective in combination than just antibiotics alone against urinary tract infections.
Simple and ancient: Ok hands up I did borrow this title from a previous blog on the built environment, but diving into ancient knowledge can also lead to solutions for AMR. Baltic amber has been used in traditional medicines as a painkiller and researchers have found a number of compounds in powder extracts from baltic amber that were active against gram-positive bacteria such as S. aureas - many strains have developed resistance to multiple antibiotics including pretty much all penicillins. A mediaeval remedy, Bald’s eyesalve, from the 1,000 year-old Bald’s Leechbook had powerful antibacterial properties.
Vaccination: As well as the direct benefit that vaccination can have on enabling our immune systems to better fight off virus-based diseases, there are also lateral benefits for dealing with bacterial pathogens. A study found that vaccinations lowered rates of acute respiratory infections by 19.7% and diarrhoea by 11.4% in under-fives resulting in a drop in antibiotic demand in low- and middle-income countries.
Microbiome: We discussed earlier the importance of having the right balance of microbes in our guts. Diet and sometimes supplements can help, but another approach is to transplant a microbiome from one person to another. Faecal microbial transplantation has been found to be more effective than vancomycin or fidaxomicin to treat C. diff infections and at a lower cost than using those antibiotics.
Behavioral solutions
To keep current antibiotics working behavioural changes are needed to reduce the need to use them in the first place.
This includes improving hygiene at personal and corporate levels to reduce the chance of getting an infection and changes to how people interact to reduce the risk of spreading bacteria. A better understanding of when and what type of antibiotic is needed to treat an infection can help reduce usage and slow down the development of AMR. This is mainly about “removing the microbe, or stopping it being there in the first place.”
John Rex who founded AMR solutions, an excellent resource for those interested in this area, has a great analogy for antibiotics. “Even when there isn’t a fire, you’re using a fire extinguisher. You bought it, you stored it, and you know it will work. Antibiotics are to infections as fire extinguishers are to fires.” We should reserve antibiotics for when they are absolutely necessary. So, what can we do?
Basic hygiene: Data from the UK Health Security Agency, published in the BMJ showed that in England in 2020 incidence of antibiotic resistant bloodstream infections fell for the first time since 2016, likely due to “changes in behaviour brought on by the covid-19 pandemic, such as less social mixing, enhanced hand hygiene, and fewer people in hospital.” Interestingly they also found that the proportion of bloodstream infections that were resistant to one or more antibiotics was the same as in 2019 and actually higher than in 2016.
Stewardship: Antibiotics are often prescribed to patients with symptoms of an infectious disease regardless of whether they are actually effective against that particular pathogen. This can be driven by a lack of testing and no requirement for prescription.
In Sub-Saharan Africa the proportion of non-prescription antibiotic requests or consultations that resulted in the supply of antibiotics was 69%. Faster and more accurate point-of-care tests (POCT) should result in more efficient prescribing of antibiotics. This in turn should help slow down the proliferation of resistance.
A 2020 study found that more than £300 million is spent on unwarranted antibiotics per year in the UK. The cost of purchasing POCTs is more than offset by savings made from reducing unnecessary antibiotics prescriptions. Acute respiratory infections account for more than two-thirds of total UK antibiotics prescriptions even though most are viral, not bacterial. The NHS could potentially save £89m per year.
The WHO recommended that patients in some cases should not continue taking antibiotics post surgery which has been backed up by a number of studies showing no difference to those patients given a placebo including this one on routine endoscopic sinus surgeries and a broad study of almost 20,00 patients from the University of Amsterdam.
Agriculture: One of the most important areas for developing better stewardship is agriculture. The FAIRR initiative (‘Farm Animal Investment Risk and Return’) was founded with the intention of driving change in the animal agriculture industry. An important aspect is the overuse of antibiotics, particularly in intensive farming. Notably in addition to treating diseases in animals it is also used in sub-therapeutic levels in concentrated animal feed to promote growth, prevention of disease (a higher risk given the closer proximity of the animals) and in improving feed conversion efficiency. Changes in the way that animals are reared can greatly reduce antibiotic consumption, for example through open grazing and absolute reduction in consumption.
Urbanisation and habitat: Studies have found that cities have their own unique microbiome and that some cities have more antibiotic-resistant genes than others. With the urban population expected to reach two-thirds of the global population by 2050, understanding this could help identify where outbreaks of diseases are more likely and target at the local level. In addition, a city’s microbiome could be a potential source for novel antibiotics. Changes to the landscape and habitat, both natural and by humans, can bring species into closer contact than they would normally be and risk the development of zoonotic diseases - we are sadly all too familiar with one of those in recent years.
Live animal markets that are culturally significant in parts of Asia and Africa are thought to be one source. Coordinated planning to adapt those markets whilst maintaining local income, trade and access to food are key. Deforestation and destruction of natural features is one other way in which species are brought together and so better planning, urban design and agricultural design, as previously mentioned, are important. A modelling study predicts that climate change will drive more than 15,000 new cases of mammals transmitting viruses to other mammals. So at the macro level, measures to address climate change are also intrinsically linked to the AMR problem.
Water systems: Misuse of antibiotics often results in excess amounts entering water systems (rivers, estuaries, deltas, etc) as we discussed earlier. This in turn can lead to resistance building in aquatic bacteria which can then enter the food chain. Therefore preventing antibiotics from entering water systems or safely and economically removing them if they do enter will be very helpful. A joint UK-India project AMRFLows is examining how antimicrobials and resistance flows from manufacturing to people through water courses. Filters and even PET bottles (or rather an extract from them) to remove antibiotics in wastewater, as well as careful dosage management (including packaging) and collection of surplus dosages can all help to reduce excess antibiotics from entering water systems. Ozone may be used to remove antibiotic and other pharmaceutical residues from water.
Physical solutions
As well as chemical compounds that can fight bacteria and other microbes, sometimes physical properties can be used to destroy or disrupt the action of harmful microbes.
Physical-based solutions, such as surface coatings, filter systems and even light and electricity can be used to make it harder for bacteria to form colonies. These solutions are mainly about “stopping microbes becoming pathogenic.” Here are some examples:
Dragonfly wings: biomimicry or the emulation of nature to solve complex human problems has brought solutions to AMR too. Dragonfly and cicada wings have antibacterial and antifungal properties. Their surfaces have tiny spikes or nanopillars which physically disrupt the membranes of the bacteria inducing oxidative stress and destroying them. They can also prevent biofilms from forming. This opens up the possibility of creating antibacterial coatings or surfaces for example for use in hospitals, filters and food packaging.
Let there be light!: A number of studies have found that blue light may be a possible physical solution for decontaminating hospital environments as well as localised infections. It works by generating reactive oxygen species by the bacteria themselves when exposed to the light, particularly when that light is at wavelengths of between 460nm to 470nm.
Ultra-low voltage: Electricity from a household battery or a small solar panel no more than a centimetre square could be used to sterilise surfaces and help water purification. The ultra-low voltage creates holes in membranes around the bacteria thereby killing them.
Materials: Certain materials possess antimicrobial properties intrinsically. The antimicrobial effect of copper has been known for centuries, and ongoing research is being conducted on the use of copper-coated hard and soft surfaces to reduce infections. Copper has been shown to be effective on both MRSA and VRE and correlated with quicker wound healing. Here is an interesting potential application - an antimicrobial second skin using copper nanomesh.
Coating and infusing: Spider silk material has been found to stop biofilms forming as well as helping human tissue to regenerate in wounds. This could lead to the development of coatings potentially eliminating the growth of biofilms on an array of items, including medical equipment. When combined with the power of current antibiotics it could be even more potent. A number of surfaces for both food preparation and medical uses can be infused with antibacterial compounds to reduce the risk of bacteria becoming pathogenic. A smart dental implant can resist bacterial growth by using a barium titanate-infused material to repel the negatively charged cell walls of bacteria.
Ozone: We already mentioned ozone being used to remove antibiotics from water, but it has also been used as a washing alternative for clothing. For example, Advanced Clothing Solutions uses ozone to kill germs and viruses including E.coli, S.aureus and coronavirus.
Financial & Political solutions
Appropriate funding opportunities can attract pharmaceutical companies to develop and bring to market novel antibiotics to treat drug-resistant infections.
In addition, for the other types of solutions already mentioned, investors can step in to fund holistic and lateral thinking innovators. Public and corporate policy changes can contribute to stronger hygiene practices across the board, along with improved health care. Lobbying is another way to target better and more responsible use of antibiotics.
Pull and push incentives: A significant challenge in bringing novel antibiotics to market is that the traditional financial incentives model does not work. Traditionally a pharmaceutical firm spends a large amount of money on R&D in the hope of recouping that spend (plus some profit for shareholders and for reinvestment) in the form of sales of the drug. i.e. a consumption-based model. The problem with antibiotics is that many are genericised and so pricing is low, but also, for good stewardship reasons, doctors are reluctant to prescribe them, hence consumption is low. Over the last 10 years, the average revenue for an antibiotic has been just $50 million which is just not enough and as a result investors had fled the antibiotics field with share prices down 50-70 percent for anti-infections companies.
Pull incentives: A value-based model should incentivise drug companies to develop antibiotics: A pull incentive. As John Rex puts it “The fire department isn’t paid per fire. You don’t buy a fire extinguisher as the fire is breaking out.” There are some examples of schemes and legislation providing a pull incentive.
The UK NHS subscription scheme, part of the NHS long term plan is designed to incentivise pharmaceutical companies to bring novel antibiotics to market via a subscription model based on the value of the product to the NHS rather than current consumption-based models. Originally announced in July 2019, two “superbug busting drugs” are due for rollout this year.
In the EU, the Transferable Exclusivity Extension (TEE) proposal could bring two new antibiotics a year to patients over the next 10 years. TEE works by providing the developer of an eligible, novel antibiotic a voucher that can be used to extend the exclusivity of one of its own medicines for a period of time, or sold to another company, thereby paying for successful antibiotic research. A cost/benefit analysis published by the EFPIA shows that individual countries could save hundreds of millions of Euros.
The GAIN Act of 2012 (Generating Antibiotic Incentives Now) which provided amongst other things an extra 5 years of nonpatent exclusivity has been disappointing. One factor has been that it disproportionately rewarded modifications to existing drugs rather than the creation of new drugs. The US Pasteur Act proposed by a bipartisan group of lawmakers was due to offer a similar structure to the NHS subscription scheme but is currently held up.
There are a number of organisations, guidance, policies and pieces of legislation that can help lubricate the system and bring finance to bear effectively on innovation. Here are some examples:
CARB-X, Combating Antibiotic-Resistant Bacteria Biopharmaceutical Accelerator (CARB-X) is a global not-for-profit based at Boston University that is funded by governments and foundations to fund early stage pipelines of new antibiotics, vaccines and other related products and focuses on the most serious drug-resistant bacteria. .
GARDP, The Global Antibiotic Research and Development Partnership is another not-for-profit organisation developing new treatments for drug-resistant infections, created by the WHO and the Drugs for Neglected Diseases Initiative. GARDP has screened 65,000 compounds and evaluated more than 100 chemical entities for antibacterial activity leading to six of those entities being integrated into GARDP’s pipeline, to be developed as potential treatments for drug-resistant infections.
The AMR action fund brings together governments, private companies as well as philanthropic organisations and gets them working together. The fund aims to invest $100m this year and $1bn over 10 years in biotechs developing treatments to tackle AMR aiming for between two and four novel antibiotics by 2030. Importantly the allocation decisions are made by an independent scientific board and NOT the investors. The first beneficiaries of the fund were announced earlier this year as Adaptive Phage Therapeutics and Venatorx Pharmaceuticals working with bacteriophages and a beta-lactamase inhibitor respectively.
Conclusion
Antimicrobial Resistance (AMR) is a macro threat to the sustainability of the human race and other species. It is of a similar level to that of the worst impacts of climate change. Bringing together biological, behavioural and physical solutions with appropriately incentivising funding we should be able to continue to enjoy the benefits of our microbe partners whilst avoiding their darker side.
We shall continue to explore this theme in subsequent articles.